
[ad_1]
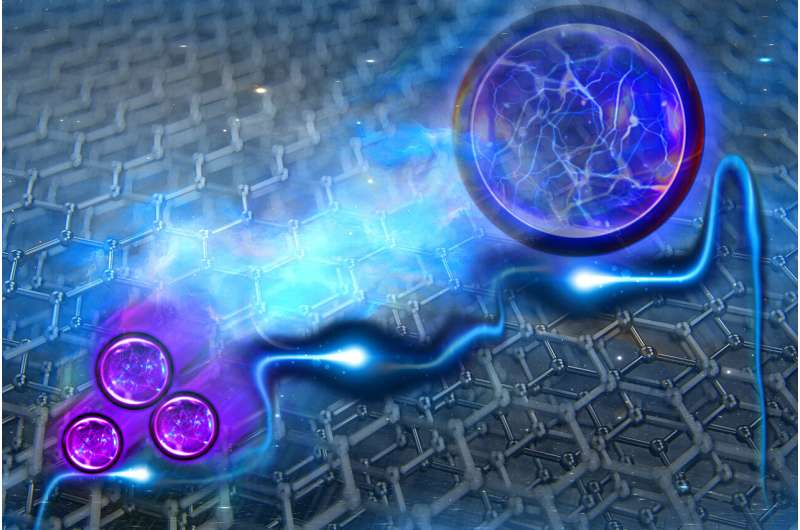
The fractional quantum Hall effect has typically been observed under very high magnetic fields, but MIT physicists have now observed it in simple graphene. In a five-layer graphene/hexagonal boron nitride (HBN) Moir superlattice, the electrons (blue balls) interact strongly with each other and behave as if they are broken into fractional charges. Credit: Sampson Wilcox, RLE
The electron is the basic unit of electricity, because it carries a single negative charge. This is what we are taught in high school physics, and it is abundant in most materials in nature.
But in very special states of matter, electrons can split into different parts of their whole. This phenomenon, known as “fractional charge,” is extremely rare, and if it can be crawled and controlled, the exotic electronic state could help build flexible, fault-tolerant quantum computers. .
To date, this effect, known to physicists as the “fractional quantum Hall effect,” has only been observed a handful of times, and mostly under very high, carefully maintained magnetic fields. Recently, scientists have observed the effect in materials that did not require such powerful magnetic manipulation.
Now, MIT physicists have observed the elusive fractional charge effect, this time in a simpler material: five layers of graphene — an atom-thin layer of carbon derived from graphite and ordinary pencil lead. They Reports Their results The nature.
They found that when five sheets of graphene are stacked like ladders, the resulting structure naturally provides the right conditions for electrons to pass through as different fractions of their total charge. which requires no external magnetic field.
The results are the first evidence of a “fractional quantum anomalous Hall effect” (the term “anomalous” refers to the absence of a magnetic field) in crystalline graphene, a material that physicists did not expect to exhibit the effect.
“This five-layer graphene is a material system where there are many good surprises,” says study author Long Ju, assistant professor of physics at MIT. “Fractional charge is completely exotic, and now we can realize this effect in a very simple system with and without a magnetic field. Which is itself important to fundamental physics. Robust against perturbations.”
Co-authors from MIT are Kenji Watanabe and Takashi Taniguchi at the National Institute for Materials Science in Japan, along with lead authors Zeng Guang Lu, Tong Hong Han, Yuxuan Yao, Eden Reddy, Jiaxiang Yang, Jun Seok Siu, and Liang Fu. are
A strange situation
The fractional quantum Hall effect is an example of the strange phenomena that can arise when particles move from behaving as individual units to acting together as a whole. This collective “correlated” behavior emerges under special conditions, for example when electrons are slowed from their normal frenetic speed to a crawl that enables the particles to perceive and communicate with each other. These interactions can produce rare electronic states, such as an apparently unconventional distribution of electron charge.
In 1982, scientists discovered the fractional quantum Hall effect in gallium arsenide heterostructures, where a gas of electrons confined in a two-dimensional plane is placed under high magnetic fields. This discovery later won the group the Nobel Prize in Physics.
“[The discovery] That was a big deal, because these unit charges interacted in a way that was very, very strange to give something like a fractional charge,” says Joe. “At the time, there were no theory predictions, and experiments. surprised everyone.”
These researchers achieved their breakthrough by using magnetic fields to slow down the material’s electrons enough for them to interact. The fields they worked with were 10 times stronger than what normally powers an MRI machine.
In August 2023, scientists at the University of Washington reported the first evidence of fractional charge without a magnetic field. They observed an “unusual” version of the effect in a twisted semiconductor called molybdenum ditelluride. The group prepared the material in a specific configuration, which theorists predicted would give the material an inherent magnetic field to encourage electrons to fractionalize without any external magnetic control. Enough.
The “no magnet” result opens a promising path to topological quantum computing—a more secure form of quantum computing, with an additional component of topology (a property that remains unchanged in the case of weak deformation or perturbation). ) qubit provides additional protection. When calculating.
This computation scheme is based on the combination of fractional quantum Hall effect and a superconductor. This used to be almost impossible to realize: a strong magnetic field is required to obtain a fractional charge, while the same magnetic field would normally kill a superconductor. In this case the fractional charges would act as a qubit (the basic unit of a quantum computer).
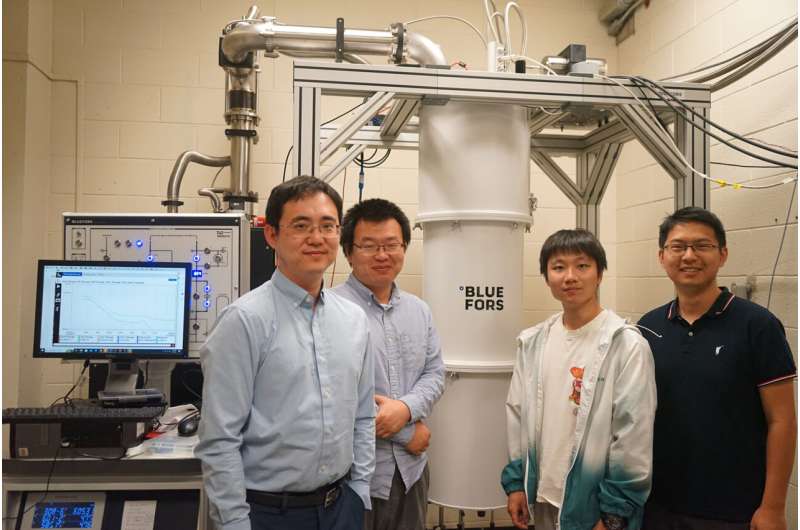
A photo of the team. From left to right: Long Ju, postdoc Zheng Guang Lu, visiting undergraduate Yuxuan Yao, graduate student Tong Hongheng. Credit: Jacksang Yang
Taking action
That same month, Jo and his team also observed signs of unusual fractional charge in graphene — a material that had not been predicted to exhibit such an effect.
Joe’s group is exploring electronic behavior in graphene, which itself has exhibited unusual properties. More recently, Joe’s group has looked at pentalayer graphene, a structure of five graphene sheets, each spaced slightly apart, like rungs on a ladder.
Such a pentalayer graphene structure is embedded in graphite and can be obtained by exfoliation using Scotch tape. When placed in a refrigerator at ultracold temperatures, the structure’s electrons slowly crawl and interact in ways they don’t at high temperatures.
In their new work, the researchers did some calculations and found that electrons can interact more strongly with each other if the pentalayer structure is attached to it. Hexagonal Boron Nitride (hBN)—a material with an atomic structure similar to graphene, but with slightly different dimensions.
In combination, the two materials should create a moiré superlattice — a complex, scaffold-like atomic structure that can slow down electrons in ways that mimic a magnetic field.
“We did these calculations, then thought, let’s go for it,” says Joe, who last summer installed a new diffusion refrigerator in his MIT lab, which the team uses to store materials at extremely low temperatures. was planned to be used for cooling. Study exotic electronic behavior.
The researchers created two prototypes of the hybrid graphene structure by first exfoliating the graphene layers from a block of graphite, then using optical tools to identify the five-layer flakes in a step-like arrangement. They then sealed the graphene flake onto the HBN flake and placed another HBN flake on top of the graphene structure. Finally, they attached an electrode to the structure and placed it in a refrigerator, set near zero.
When they applied a current to the material and measured the voltage output, they began to see the signature of fractional charge, where the voltage equals the current multiplied by a fractional number and some basic physics constants.
“The day we saw it, we didn’t recognize it at first,” says first author Lu. “Then we started screaming as we realized, this was really big. It was a completely amazing moment.”
“This was probably the first serious sample we put in the new refrigerator,” says co-first author Hahn. “Once we calmed down, we looked in detail to make sure what we were seeing was real.”
With further analysis, the team confirmed that the graphene structure did indeed exhibit the fractional quantum anomalous Hall effect. This is the first time the effect has been observed in graphene.
“Graphene can also be a superconductor,” says Joe. “So, you can have two completely different effects in the same material very close to each other. If you use graphene to talk to graphene, that leads to a lot of unwanted effects when mixing graphene with other materials. survives.”
For now, the group continues to explore the multilayer. Graphene For other rare electronic states.
“We’re diving in to explore many of the fundamental theories and applications of physics,” he says. “We know there’s more to come.”
More information:
Long Ju, Fractional quantum anomalous Hall effect in multilayer graphene, The nature (2024). DOI: 10.1038/s41586-023-07010-7. www.nature.com/articles/s41586-023-07010-7
Provided by
Massachusetts Institute of Technology
This story is reprinted courtesy of MIT News (web.mit.edu/newsoffice/), a popular site that covers news about MIT research, innovation, and teaching.
Reference: Electrons become fractions of themselves in graphene, study shows (2024, February 21) Retrieved February 21, 2024 from https://phys.org/news/2024-02-electrons-fractions-graphene.html has been
This document is subject to copyright. No part may be reproduced without written permission, except for any fair dealing for the purpose of private study or research. The content is provided for informational purposes only.
[ad_2]